Standing Structures
Scarcity Zero’s first target for large-scale renewable deployment are standing structures with a primary emphasis on solar power. As we’ve invented solar panels that are both clear and visually unobtrusive, virtually any type of construction can be harnessed to generate clean energy from the sun.
This approach is ideal when it comes to location for solar deployment: standing structures are directly integrated within (and thus close to) population centers, and, as such, don’t require the purchase of additional land that might otherwise cost billions of dollars in aggregate. Further, in many cases, integrating solar can be accomplished as a seamless upgrade of the building’s existing features.
As a base concept, this idea isn’t new. Rooftops have been outfitted with solar panels since they were invented, and their use has increased as the costs of renewable energy have dropped over time. But recent advances in manufacturing and material science empower us to more deeply integrate solar into architectural designs. Solar glass now allows buildings to replace exterior windows with transparent solar panels. Solar-thermal HVAC uses excess solar energy to heat water and the building itself, generating electricity while at the same time slashing energy costs. Rooftop solar panels have never been cheaper, and for residential applications, solar shingles give homeowners the ability to use solar power with zero aesthetic sacrifice. Next-generation energy storage technologies tie all of the above together in a reliable, closed-loop system – an important feature, which we’ll review later in this writing.
When applied to existing buildings on the scale of skyscrapers or large office complexes, these advances can easily reduce energy use several times over – if not turn commercial buildings into mini power plants. To explain how, let’s circle back to windows. Consider the skyscraper to the left for a moment. Now imagine if every single one of those windows were replaced with solar glass – which again can be made aesthetically identical to normal glass. Just for one skyscraper, the energy potential would exceed hundreds of thousands of kilowatt-hours, even at moderate efficiencies – which would account for shadows or cloudy days as solar still works under such conditions. [13]
According to SolarWindow, a leading manufacturer of solar glass for commercial applications, a 50-story skyscraper in downtown Manhattan would generate 1.12 million kilowatt-hours annually if completely outfitted with solar windows (equivalent to the annual electricity usage of 108 single-family homes).[14] That number would rise to 1.38 million annually in Denver, and rise further to 1.57 million in Phoenix.[15] With present energy costs, the time it would take for each of those installations to pay for themselves is estimated to be roughly one year.[16] As solar glass can auto-tint based ambient brightness, the potential dividends for insulation and indoor climate management can be extended further.
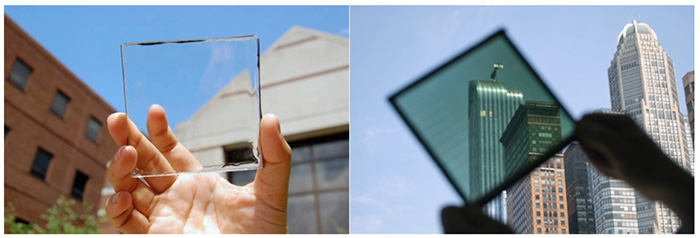
A large building such as the skyscraper above could likely generate more energy than it could reliably consume in a given day. What to do with this extra energy? It could be held locally in municipal storage (including the National Aqueudct), sold back to a local or municipal electric utility, or it could be diverted directly into its heating and cooling system.
The image to the right shows a basic diagram for a solar-thermal heating system. In concept, excess solar energy is used to heat water during the day, which is kept warm in an insulated storage tank. As water holds its temperature better than nearly any other substance, solar-heated water stored in insulated tanks dramatically reduces the need to use oil or natural gas for building heat. Notably, as solar panels are most efficient at colder temperatures,[17] this can save companies significant sums through reduced heating bills.
The benefits of upgrading existing buildings with solar windows and rooftop arrays of solar power will be limited primarily by the construction of the buildings themselves. To truly extend the capabilities of renewables on standing structures, integration could be considered by design at the architectural stage.
The following image shows the circular headquarters for PAF, a gambling management company owned and operated by the Finnish government. The building is wrapped completely in solar panels.
Another example is the Solar Valley Micro-E hotel in Northwest China, designed from the ground-up to maximize solar utility.
The Sanyo/Panasonic Solar Ark is a dual power plant and science museum dedicated to solar energy. Made from factory-rejected solar panels, it generates 500,000 kilowatt-hours of energy per year.[18]
These examples of solar buildings are prototypes, and, with the exception of the PAF headquarters in Finland, are initiatives undertaken by private companies. But these buildings act as solid proofs-of-concept, giving us ideas with which we can extend the integration of renewables within new structures.
Unlike passive solar design – an architectural trend from the 1970’s that attempted to design structures that would naturally take advantage of solar energy without the aid of mechanical equipment[19] – the sophistication of today’s technology and available commercial products in the renewable sector make “active solar designs” more straightforward. Technology can provide solar shingles, solar windows and solar walls at comparable or lower costs than fossilized energy sources.[20] Such technologies can further integrate through solar-enabled heating and air conditioning systems within a building. Managing a system could be accomplished through something as simple as a smartphone app.
Approaching architectural design with an eye to using active solar saves significant sums of energy and money. According to the U.S. Energy Information Administration, roughly 25% of a given commercial building’s total energy use was devoted to heating in 2012,[21] consuming the equivalent of some 556 billion kilowatt-hours of power in aggregate. At a rate of 10 cents per kilowatt-hour, that’s equivalent to $55.6 billion just to keep buildings heated – saying nothing of the electricity costs to keep the lights on and run equipment.
Every building that adopts an active solar system avoids unnecessary energy expenses and further presents less demand on the local electrical grid. Each building further helps create a cascading effect of increasing the amount of energy generated while decreasing total energy consumption.
This cascading effect becomes important when other infrastructure is selected for potential solar energy deployment. Take bridges for example.
The Sunshine Skyway Bridge in Tampa, for example, is 4.14 miles long (roughly 21,900 feet).[22] If we were to assume an average of only 40’ of deployment surface on each side of the 430-foot tall structure, the Skyway bridge would feature 1.75 million square feet of surface area that could be mounted with clear, aesthetically unobtrusive solar panels. When we shortly consider the implications of solar road canopies, the 94’ wide driving surface would add another 2.05 million square feet of surface area to a total of 3.81 million.
As one square foot of solar panels can reliably generate 30 kilowatt-hours annually in areas with solid sun exposure, the Skyway bridge could passively generate 114 million kilowatt-hours of electricity per year without having to buy a single piece of land – enough to power nearly 11,000 homes.
Another promising example of usable infrastructure is the parking lot. Our nation is littered with parking lots that are flat and open:
As they’re dark in color, they absorb lots of heat, and anyone who drives in summer knows full well that they turn cars to ovens – presenting serious safety risks to children or pets left inside. This makes parking lots uniquely well suited for solar panels, a concept that’s been increasingly adopted as solar panel prices have dropped.
Solar parking lots solve several problems at once. They generate electricity that can be used to power the building that owns the lot; charge the electric cars of customers or employees; or sell energy back to a local or municipal electric grid. Solar canopies further provide shade that keeps cars cool. Across an urban landscape, solar parking lots could generate a massive amount of electricity that could further increase our total generating capacity (and thus supply) while reducing demand from external energy sources.
Assuming a parking lot of 150,000 square feet (roughly equivalent to a local Lowe’s),[23] sticking to the figure of 30 kilowatt-hours of electricity generated per square foot, per year, that parking lot would generate roughly 4.5 million kilowatt-hours annually. If for sake of argument, the local Target, Home Depot, Walmart, Costco and Sam’s all had the same footprint, the combined total would be 27 million kilowatt-hours annually generated. That’s enough to power more than 2,500 homes. It’s plain, then, to see how these numbers can add up quickly over large areas of urban sprawl. This becomes even more true once road medians and solar road canopies enter the equation.