Up until now, this writing has primarily focused on the technologies Scarcity Zero proposes to solve resource scarcity. The reason for this is unambiguous: resource scarcity is the core human malady. It’s the primary cause of large-scale conflict, the major driver of environmental destruction and climate change, and both the facilitator and accelerant of economic decline, poverty and state-level enmity. Scarcity has been a determining factor in our existence since civilization became civilization. It’s solution, therefore, is the central factor in determining our evolution beyond the dynamic that’s limited us from that time-onward.
The question thus becomes: what happens once we reach that zenith?
Concretely, we’ll have a game-changing abundance of resources. Resources sourced not only from next-generation and highly scalable technology, but also from freeing up immense resources that were previously devoted to mitigating the consequences of scarcity, both at home and abroad. Mainstays of state spending will experience a paradigm shift: defense, security, finance, agriculture, manufacturing, energy, healthcare, construction, communications, education and beyond will all be forever changed by such abundance.
This cyclical effect has the potential to reshape our future and improve our quality of life on a scale unrivaled. This goes beyond thinking bigger and building larger. The very foundations of our civilization will be on a trajectory of collective ascent to heights that were never before possible until we reached this threshold.
To put that statement in perspective, recall that humanity has existed for about 200,000 years, although some estimates say it’s as long as 300,000.[1] For 95% of that timeline, we were basically cavemen. If we characterize “actual” civilization as the start of the Bronze Age, that period started only 5,000 years ago. From the year 200,000 B.C.E. until the mid-1800s, the fastest a human could travel was on horseback. Yet by the start of the 20th century we had the train, automobile and aircraft, and we landed on the moon less than 70 years later. The light bulb, internet, cellphone, computer, skyscraper, satellite and spacecraft were all invented in roughly the past 1/2,000th of our history.
We achieved each of those advances through technological ascension, and advances that were limited only by our ambition of vision and our knowledge present at the time – ever-accelerating the former through advances in the latter.
Scarcity Zero accelerates our rate of ascension by providing effectively unlimited energy and resources in which to build practically anything to a superior civilizational scale and sophistication. In doing so, we are presented with unique potential to advance our social infrastructure – especially within areas of civil engineering, transportation and aerospace.
Civil Engineering
Social infrastructure – what we can build, how we build it and how long it lasts – makes our society possible, and beyond that, makes it enduring and inspiring. Scarcity Zero can revolutionize our recently-neglected social infrastructure to great social benefit by allowing public works projects to complete faster, less expensively and on larger scales.
You’ll recall that repairing the decaying infrastructure across our nation is expected to cost several trillions of dollars with today’s tools and methods – even in the most conservative estimates.[2] These are repairs that need to be made, yet we have neither the earmarked funds nor the political willpower to pay for them. However, as with energy and resources, technology provides an opportunity to solve the problem for us by leapfrogging limitations cost-effectively.
How exactly? First, let’s cover some givens:
Most heavy machinery today is powered by diesel, which makes fuel a considerable expense of any construction project.[3] And while diesel engines have legendary reliability, the pumps, belts, and hydraulics in heavy machinery aren’t generally as dependable, which leads to delays and additional costs when they eventually fail. Electric construction equipment on the other hand is mechanically simpler, and as such can avoid many of these complications while delivering the same standard of performance as their diesel counterparts.[4] As prices for electricity drop with the implementation of Scarcity Zero, fuel becomes less of a construction expense (all the more so once hydrogen becomes a more viable industrial fuel).
Building materials also command significant percentages of construction budgets.[5] With Scarcity Zero, construction projects could source better materials for lower prices. This would enable us to build lighter and stronger structures with less expense than we can today; and as a structure’s maximum size is limited largely by strength-to-weight ratios, these materials would also increase the scale of what we are capable of building.
Once computer modelling, 3D printing, and factory prefabrication are added in, however, is when we truly start building the future. These technologies have been around for only the past decade, meaning that the majority of structures in our society were built without the aid of computers, and anything built before the late 1970s didn’t even involve a calculator. Today, architectural software allows us to design structures virtually on computers. This provides engineers with 3D representations of what they’re constructing along with highly accurate predictions of material requirements and limits of load and scale. The following images, for example, respectively show a bridge being designed on a computer and another being 3D printed in real time:
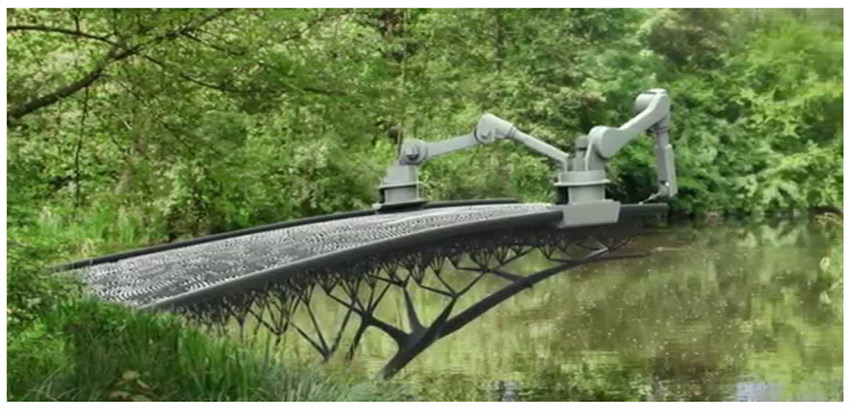
Considering that many of these technologies are in their infancy with regards to civil infrastructure, there is ample room for them to grow in the future. Integrating improvements in material science, nigh-unlimited energy and resources, and design-first principles of standardization and modularity only stand to serve as accelerants. We previously saw how we can embrace these advances to build houses, buildings and prefabricated systems, but this concept applies to the manufacture of practically anything on a large scale.
If we can prefabricate jetliners and LFTRs, why not bridges, tunnels, apartment buildings, and skyscrapers? Aerospace-grade engineering carries the highest requirements for reliability in the world, and today we already can completely assemble a flagship jetliner every nine business days – or 3D print sophisticated components for the same in a matter of hours. A world powered by Scarcity Zero grants us the means to raise the bar higher still.
Within civil engineering, examples include:
Next-generation roads, bridges and tunnels. Railroads and paved highway networks rank among the greatest marvels of human engineering, revolutionizing travel, transportation and commerce on global scales.
Yet in many ways, such infrastructure is only as useful as its ability to overcome obstacles in the landscape, something made possible through bridges and tunnels – engineering accomplishments that we don’t often think about when impressive structures come to mind. And yet:
- While not the longest bridge in the United States, at 4.8 miles the Chesapeake Bay Bridge is one of the most important, as it connects Delaware and Maryland’s Eastern Shore with the Baltimore-Washington Metropolitan Area. Roughly 25.6 million vehicles travel on it every year, each one saving time and fuel that would be devoted to longer routes should the bridge not exist.[6]
How much time and fuel? Assuming each of these 25.6 million vehicles traveled between Washington, DC and Dover, Delaware, they need drive only 93 miles for 1.8 hours if they use the bridge. If not, they would need to drive 134 miles for 2.75 hours via I-95.[7] This means that over the past 10 years, assuming consistent traffic and 21 miles per gallon fuel economy, the Chesapeake Bay Bridge has collectively saved motorists a total of one billion miles of driving distance, 224.3 million hours (2,776 years) of driving time, and roughly 500 million gallons of fuel.
- The Colorado I-70 corridor splits the Rocky Mountains with a highway, allowing motorists to avoid slow and often precarious mountain passes. The corridor is made possible through the 1.7-mile-long Eisenhower-Johnson tunnel, which was completed in 1979. It takes approximately four hours on I-70 to travel the 235 miles from Denver to Grand Junction on opposite sides of the Continental Divide. Without the corridor, it would take approximately 8.6 hours to travel the 432 miles via U.S. Route 40.[9]
To put those numbers in perspective, the highway and tunnel has saved each vehicle 4.6 hours of driving time and a driving distance of 197 miles. As roughly 13 million vehicles travel through the tunnel annually,[10] we’ll conservatively assume that from 1979 to 2018 a total of 400 million vehicles have traveled this route to Grand Junction. At an assumed average fuel economy of 21 miles per gallon, this tunnel system has collectively saved drivers 79 billion miles of driving distance, 1.8 billion hours (210,000 years) of driving time, and 3.7 billion gallons of fuel.
Under those assumptions, these two public works projects, alone, have collectively saved motorists a total of 80 billion miles of driving distance, 213,000 years of driving time, and 4.2 billion gallons of fuel.
Both the Chesapeake Bay Bridge and the Colorado I-70 corridor were built with technology from the 1950s-1980s – a far cry from what we have available today, which in itself is a far cry from the capabilities we would have with Scarcity Zero. Under the framework, we would be able to increase the scale of our bridges and tunnels – connecting places in ways that were never before possible.
Megabridges: as the name suggests, a megabridge is a bridge of large size and scale. Built with the strongest materials available, a megabridge spans longer distances and support more lanes and thus heavier loads. They can also enable travel of both road and rail, increasing diversity of use and overall social utility.
Megabridges have already made their debut on the world stage. The above concept images respectively show the proposed Fehmarn Belt Fixed Link,[11] connecting Germany and Denmark, and the Sheikh Rashid bin Saeed Crossing megabridge in Dubai.[12] The image below shows the recently completed Zhuhai-Macau Megabridge connecting mainland China to the island of Macau:
As impressive as they are, neither of these projects can yet utilize large-scale factory prefabrication or next-generation synthetics, meaning they are ultimately constructed ad-hoc with less advanced material options than would be possible with Scarcity Zero. Future megabridges can avoid these constraints. Imagine if construction crews didn’t need to pour concrete, lay cable, steamroll asphalt or spot-weld junctions by hand, each and every time? What if they instead could take prefabricated pylons, platforms, support arches, and integrated renewables, assembling the entire bridge like a hobby kit, just on a larger scale?
This approach is not only possible, it can be a hallmark of tomorrow’s manufacturing capabilities. Such an approach further allows architects to expand their vision, as it reduces several of the problems with modern construction and material sciences. As civil engineers can hash out the technical details of a bridge with ever-more sophisticated software at the design stage, it could be rapidly determined what it would take to enlarge the bridge to greater scales of size, should the materials and manufacturing methods be present. Such efforts could lead to a day where bridges eight to twelve lanes wide with lengths upwards of 100 miles or more enter the realm of possibility. That is the future made possible by effectively unlimited energy and resources.
Megatunnels: the megatunnel is the evolution of subterranean / underwater transportation structures. Of the megatunnels in existence or in planning stages today, perhaps the best examples are the 30-mile Channel rail tunnel connecting England to France, the 33-mile Seikan tunnel connecting the Japanese islands of Honshu and Hokkaido, and the 35-mile Gotthard Base Tunnel under the Alps.[13]
These tunnels are rightfully considered among mankind’s most impressive accomplishments. But challenges remain to increasing their scale, especially in the context of submerged tunnels. There are unique complications to building submerged tunnels that are not present with bridges, namely the presence of extreme water pressure.
Building a submerged tunnel between England and France, for instance, is possible today, as the depth of the English Channel doesn’t exceed 150 feet.[14] Yet building a submerged tunnel from, say, Tokyo to Beijing, or London to New York, is far more difficult. When water depths reach thousands of feet, pressures are so great that hardened steel structures can crumple like paper bags. The material advances made possible by Scarcity Zero allow us to significantly extend our capabilities to build structures that can withstand such pressures. They also allow us to reach an even more achievable goal of floating megatunnels that would serve the same effect as deep water variants in function.
Through a combination of buoyancy control mechanisms or cables/weights tethering the tunnels to the ocean floor, these tunnels would stay close to above-water atmospheric pressures to avoid complications with structural integrity or surface breaches in the case of emergencies:[15]
Two concepts shown below:
As a structure’s weight displacement is different when submerged as opposed to on land, the buoyancy of these tunnels can be calibrated to maintain high degrees of stability, strong enough to support vehicle and even high-speed rail travel. Norway is already considering building submerged tunnels to cross fjords, a model that could be applied to more ambitious projects over larger bodies of water as our technology improved.[16] Scarcity Zero-underwritten energy cost and material advancements bring this possibility closer to reality.
Luminal communication networks. While perhaps not to grandiose scales in physical terms, the information networks we have built over the past three decades rank among the most advanced infrastructure in history.[17] In the United States, however, these networks are becoming ever-more dated. While corporate monopolies and broken politics certainly don’t help,[18] the primary problem is one of distance. The vastness of the United States presents challenges to providing high-speed internet nationwide at low cost – costs that have to be paid over and over again once outdated technology needs to be updated.
As we saw throughout much of this writing, municipally integrated renewables and the National Aqueduct provide ample opportunity to run utility lines, including those for communication. This gives us a natural platform to run internet cables over any distance effectively, as their generated electricity could power amplification systems to prevent signal loss. And instead of traditional cables that transfer data through copper wires, we can now install fiber-optic cables that are 30-100 times faster.[19]
If internet service were embedded throughout renewable-integrated highway networks and the National Aqueduct, we’d effectively turn the country into a giant antenna. This process further becomes more cost-effective, because running fiber cables through above-ground conduits is far easier and less expensive than today’s method of running cables underground. If municipal internet were provided through road networks and the National Aqueduct, we would effectively have nationwide wireless internet, low in cost an expansive in scale – cementing a next-generation information backbone for everyone in society.
Prefabricated buildings. We’ve so far talked at length about the concepts of prefabrication and 3D-printing, with attention to how we can use them to build advanced systems quicker, better, and with less expense. Megatunnels and megabridges are good examples of how we can apply these concepts to larger-scale infrastructure, but there are also other promising applications – such as extending residential prefabrication beyond what we saw last chapter. The following three images show the “One9,” a nine-story prefabricated apartment building in Melbourne, Australia that was installed in just five days.[20]
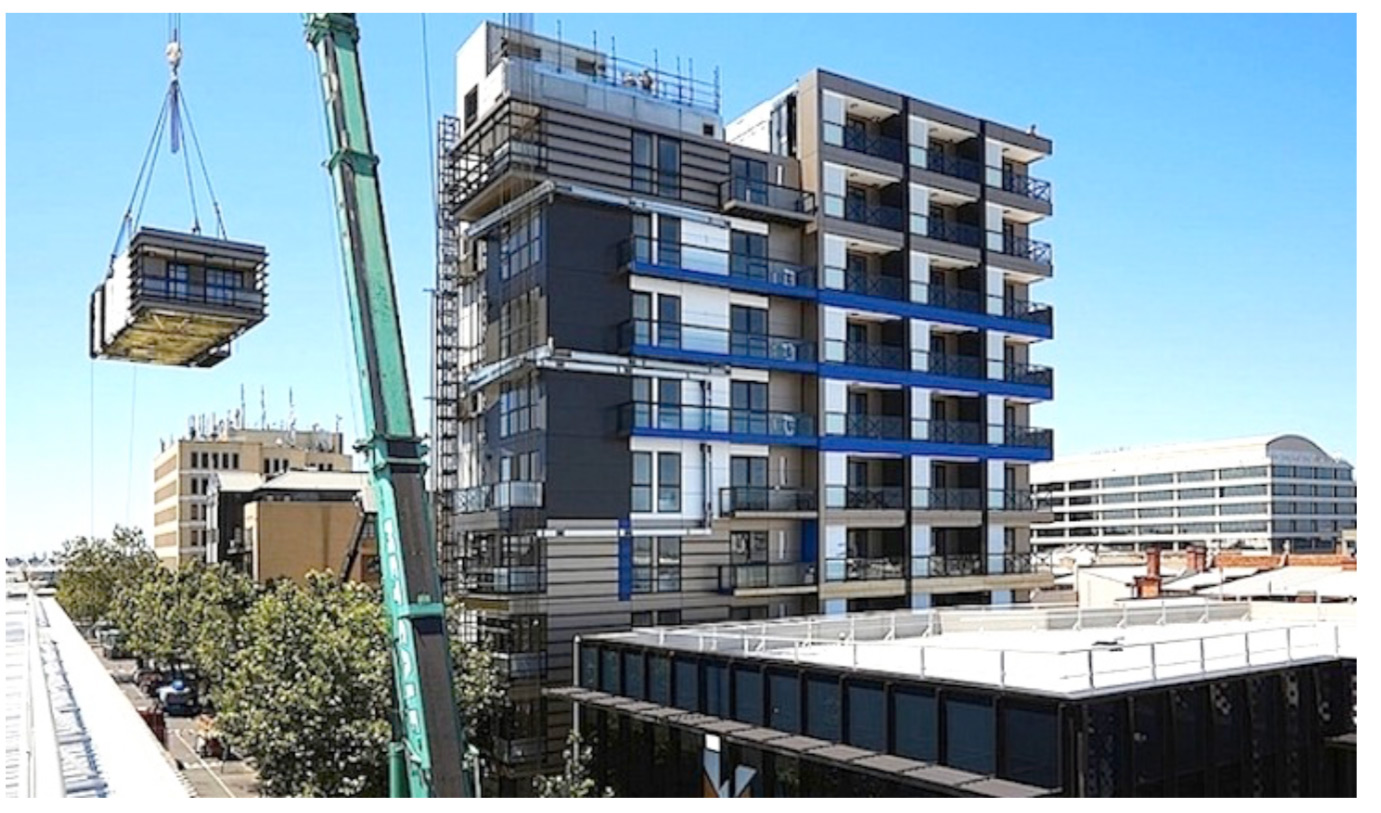
This apartment complex in Kansas City features 80 modular units that was finish-assembled on-site within four months:[21]
For comparison, the average time to construct a single-family home in the United States is between 6-11 months, once a building permit is issued.[22] Prefabricated structures such as these are attractive options for reducing housing shortages, a problem that is expected to increase as large numbers of people continue to migrate to cities.[23]
Prefabrication also works on even larger scales. We saw in Chapter Eleven how China’s Broad Sustainable Buildings assembled a 30-story tower in 15 days. But that’s only a pioneering example of the potential of prefabricated structures. The company has since outdone themselves by building a 57-story skyscraper in nineteen days, which, at three stories per day, is 33% faster than their previous performance. Named “J57 Mini Sky City,” the structure is one of the tallest modular buildings in the world.
Back in the United States, Skanska, a Swedish construction company, has recently completed “461 Dean,” a 363-unit apartment building in downtown Brooklyn. The 32-story building, completed in approximately 18 months, saved 20% on construction costs when compared to traditional building methods.[24]
These cost savings are then passed on to prospective tenants, as the studio units in this building start at $560/month in a neighborhood where the median rent is roughly $2,700.[25]
When we consider the reduced costs and shorter construction timelines on these buildings, it’s important to be mindful that they represent the first variants of this emerging technology. Further, these feats of engineering are also performed with today’s technology and material limitations. If we think about how far we've come in other areas over just the last 20-30 years, there's no telling how much more advanced this type of construction can become in the future – especially if the advances of Scarcity Zero were incorporated.
Supercities
Prefabricated, standardized, and modular construction has incredible potential to revolutionize how we build things, allowing us to raise structures far larger and far faster than we can today. When taken with the other advancements of Scarcity Zero, this stands to transform humanity’s approach to cities, and how we live in them.
As cities have evolved, they have expanded in population and sprawl, drawing in people by the billions to their economies, amenities and culture. Modern technological advancements in infrastructure, however, didn’t exist until the early-to mid-1900s, even in affluent areas. Today, even the most modest city dwellings have amenities such as running water, plumbing and electricity, as well as means of transportation and communication that would have been unthinkable for the past 99.99% of human existence. Further advances in technology can take us to an even higher stage of city living: “supercities.”
Conceptually, a supercity is an urban center that provides residents with an unprecedented quality of life by leveraging next-generation technical capability. As it has no fixed definition within our lexicon, we’ll define a supercity as having the following six criteria:
- Population: a supercity has a total population of 10 million or greater, or the ability to readily scale to support that population. This is the only requirement a supercity shares with a “megacity,” presently defined only by having a population of greater than 10 million people.[26]
- Energy and resources: although integrated with external power grids and resource production systems, a supercity is able to produce the majority of its energy and resources through municipally integrated renewable infrastructure, supplemented by external LFTRs or CHP Plants.
- Utilities as public provisions: taking advantage of inexpensive energy and simplified installation of utilities through renewable-integrated roads, a supercity provides electricity, water, heat, and high-speed internet as publicly funded municipal services.
- Advanced construction: building new structures and upgrading existing ones are top priorities for supercities. Buildings, bridges, and tunnels are rapidly constructed using prefabricated, modular methods with high energy efficiencies, and are further integrated with renewable technologies. Supercities, therefore, have a high percentage of new, modern buildings and infrastructure.
- Transportation: a supercity features advanced transportation technologies, such as maglev rail and autonomous vehicles. These are discussed in the next section of this chapter.
- High quality of life: a supercity provides excellent education, healthcare, employment and recreation at low cost with a high quality of life index.[27]
Life in a supercity would stand in stark contrast with today’s urban environments, where most areas range in quality from fantastic to poor, usually with good to mediocre mixed somewhere in between. But polarized distribution of wealth becomes less of a social malady if all necessities of life can be inexpensively provided through technology.[28] Higher-quality amenities would become more affordable, enabling everyone to increase their quality of life without necessarily having to spend more money. Essentially, Scarcity Zero would allow things to be built to the quality of the fantastic at the cost of the modest. This capability supports businesses, venues, attractions, and thus jobs – allowing any given area of a city to thrive, and in turn, advance.
As a result, everyone is afforded a greater sense of community, which translates to reduced crime and a collectively greater life experience. Utilizing this approach in all areas of a given city raises the floor and in turn enables a city to devote greater amounts of resources to continually advance, improve and evolve.
Supercities are closer to reality than one might think. Cities have been rapidly developing over the past 100 years, and tomorrow’s technology is only going to accelerate that pace. For example, take a look at the New York City skyline over the past century, starting from 1914:
In this 100-year period – a blink of an eye in historical terms – we see that the New York City skyline has grown immensely in both scale and sophistication. With the technical breakthroughs Scarcity Zero provides, we can advance urban construction at proportionally similar or reduced costs. As Scarcity Zero would enable us to prefabricate and rapidly construct effectively any type of urban infrastructure, we can grow cities to scales that are not yet possible today.
The question now becomes: what does the skyline of New York City, or any, look in a world powered by a dynamic of effectively unlimited energy and resources, 20, 50, or even 100 years from now? Futuristic concepts notwithstanding, they nonetheless represent the potential futures made possible by the advanced technology on our near-term horizon.
This is especially important because as humanity’s population is rapidly expanding, billions of people are expected to flock to cities within the next few decades.[29] For reference, roughly half of the planet lives in cities today. By 2050, that number is expected to exceed 70%.[30] Such environments must be able to scale in size in order to accommodate this shift, and supercities can do so while also supporting internal energy and resource production, advanced systems of transportation and rapid construction of social infrastructure.
Next-Generation Transportation
Until the invention of steam power, the only options for moving people or goods over distance were either horses or sailboats. Today, we have cars, trains, and planes that can carry us thousands of miles across the planet, some in a matter of hours – advances that are fewer than 100 years old. Future improvements in technology will make the world even more accessible, saying nothing of what lies beyond our terrestrial home.
One of the first of such improvements is already here: the production of vehicles and mass-transit systems that run on sustainable fuels, which Scarcity Zero helps extend through electricity, hydrogen and graphene. But in a world with nigh unlimited energy, sustainable resources, advanced manufacturing methods, and synthetic materials that are both lightweight and ultra-strong, the possibilities multiply to the limit of imagination. Some notable standouts include:
Ultra-efficient, self-driving vehicles. The past decade has made substantial headway with autonomous vehicles that are able to drive themselves without any human interaction. They work via arrays of sensors and short-range laser-enabled radar (LIDAR) that instantly relays data – like road direction, location of other vehicles, obstructions, and weather conditions – to the vehicle’s computer, which handles the actual driving and steering.
As this data is processed instantaneously, the vehicle reacts instantaneously as well – much faster than human reaction times. Autonomous vehicles are then exceptionally safe, especially since they are programmed to follow speed limits and obey the rules of the road.
One of the most extensive autonomous vehicle programs in the nation is run by Google (“Waymo”), although Tesla, Audi, Uber and several other car manufacturers have made significant investments in driverless technology.[31] Google’s program has completed over 700,000 autonomous-driving miles with 12 separate vehicles, with only one safety incident that was caused by human error.[32] Uber’s model has been slightly less successful, with one fatality deemed unavoidable due to a lone pedestrian jaywalking.[33] To compare this safety record with human drivers in the United States: 268 million vehicles annually drive 3.17 trillion miles per year[34] and are involved in roughly 10 million accidents, averaging to one accident per every 26.8 vehicles or every 317,000 miles driven.[35]
In comparison, the safety record of autonomous vehicles is a dramatic improvement. The implications of this achievement are especially important because the 10+ million auto accidents occurring annually claim the lives of roughly 33,000 people and injure some two million others.
While safety is the most important factor when considering the benefits of self-driving cars, it’s not the exclusive selling point:
- As all speed limits and road rules are obeyed, self-driving cars are highly efficient, as they can maintain a uniform speed without having to constantly accelerate or decelerate in reaction to other vehicles (assuming all others on the road are also autonomous). This makes self-driving vehicles more energy efficient and reduces traffic congestion.
- Of the 33,000 road fatalities every year, nearly a third of them come from drunk drivers; presumably the same is true of the 2.6 million injuries that occur annually as well. Self-driving cars make this problem go away effectively overnight.
This is merely the state of current technology. If Scarcity Zero’s advancements are applied, our capabilities increase accordingly. For example, instead of just having self-driving vehicles track road surfaces through internal sensors, they can also use the built-in Wi-Fi of renewable-integrated road surfaces to navigate, providing system redundancy and security. As such vehicles would increasingly be electric, they could also be charged on roads through wireless emitters embedded within municipal infrastructure. In the increasingly unlikely event of an accident, emergency crews could be instantly notified with automated reports of the extent of the damage and the number of passengers injured.
Large-scale ground transportation. The most effective way to transport goods or people over ground is rail, and the latest versions are known as “maglev” – short for electromagnetic levitation.[37] While much of the developed world has already employed this technology, America is far behind. There are several reasons for this state of affairs: the petroleum lobby, the geographical size of the United States, the extent of personal vehicle ownership, and the expansiveness of the interstate highway system.[38] But it’s time for America to embrace the future, and when it comes to large-scale transportation, maglev rail is the future.
As maglev propulsion is nearly frictionless, maglev trains can travel at speeds exceeding 300 miles per hour (482 kilometers per hour). With Scarcity Zero, building prefabricated, modular train cars and track systems becomes more straightforward. This can enable us to build trains near renewable-integrated roads or the National Aqueduct – creating an insulated mass transit system with constant connectivity to power sources. And if these trains were built on prefabricated pylons next to highways, it would also remove the need to purchase additional land for their construction, further reducing costs.
Providing improved nationwide rail networks that can transport people or goods at 300 miles per hour is a four-fold improvement over most domestic rail technology. The implications this presents for trade, transport and tourism stand to return extensive economic benefits, all the more so if considering the new job opportunities opened by America gleaning expertise in this market sector.
Yet even though such speeds are impressive for a train, maglev technology can theoretically propel trains much faster. The biggest obstacle to doing so is air resistance, which, at high speed becomes especially significant for safe operation. A system called the Hyperloop may well enable us to change that.
Hyperloop. Originally envisioned by PayPal, Space X, and Tesla founder Elon Musk, the Hyperloop is a theoretical extension of the tubular transport systems used in banks, where a capsule rides a wave of air inside a tube from one location to another. With the Hyperloop, instead of transporting a capsule it would instead transport a train, integrating maglev technology for high-speed travel.[39]
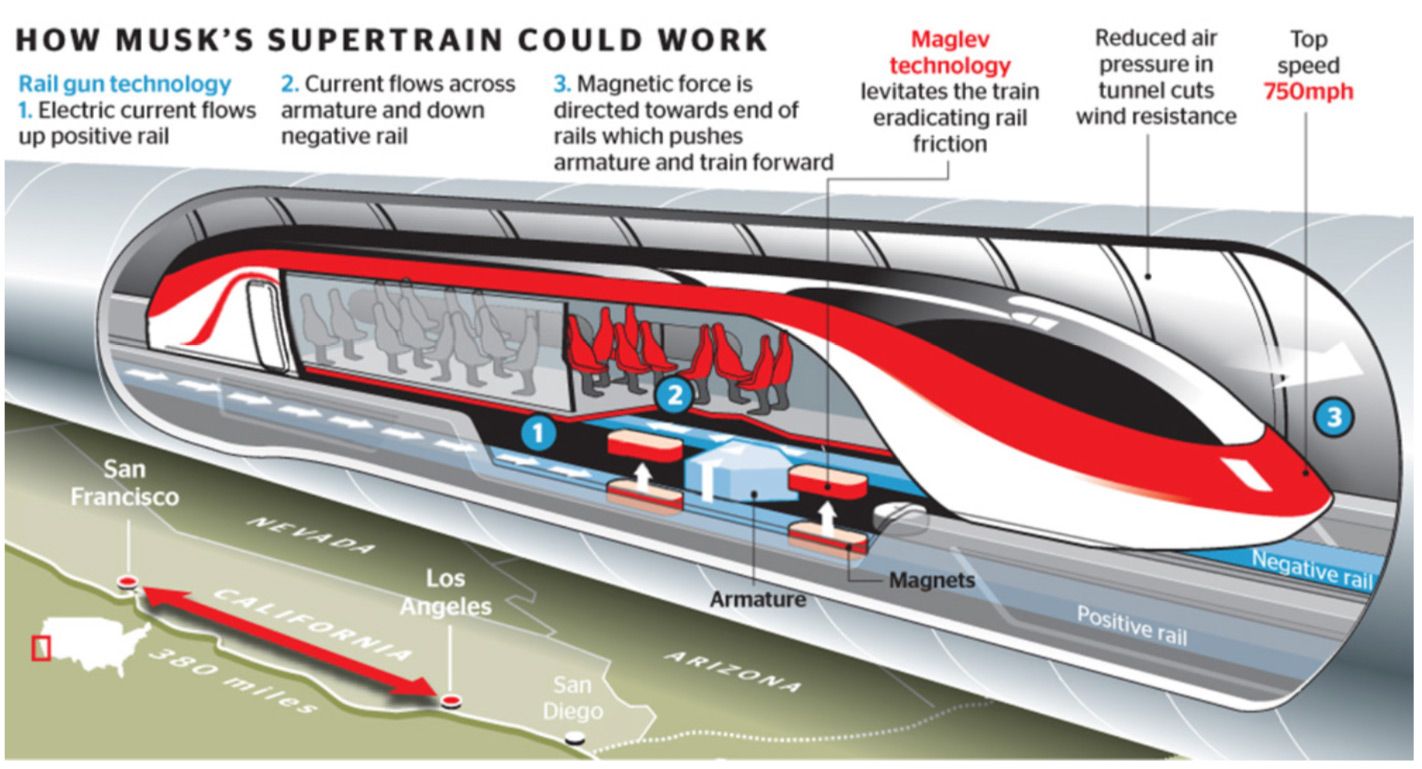
As with submerged megatunnels, the Hyperloop would operate in a partially depressurized environment. Mounting a high-strength air compressor at the front of the train would remove forward-facing air resistance and, at the same time, provide a frictionless air cushion around the train body.[40] Reduced air pressure translates to reduced air resistance, permitting the Hyperloop to travel at far higher speeds than currently possible, with reduced effects of breaching the sound barrier due to a lack of air density.
The Hyperloop concept is envisioned to be prefabricated and built on pylons by design. Connecting Hyperloop technology with integrated renewables or the National Aqueduct would also provide constant power the system as a whole, which, if built with advanced synthetics, would be stronger and lighter than most commercially available materials today.
The Hyperloop has already started construction and has demonstrated initial successes in early tests and national competitions.[41] Several companies have since emerged to build functional models in Dubai, California and Europe.[42] A world with nigh-unlimited energy and resources alongside easy, sustainable access to high-performance materials only stands to accelerate the development of next-generation technologies and their arrival to market – presenting yet another transformational addition to capabilities of human movement.
Personal Flight. The price of passenger vehicles has steadily dropped over time, making them affordable to most Americans. Airplanes, however, remain unaffordable to the majority of people, even though they’ve been around nearly as long. For a few thousand dollars, you can get a working, used car. The cheapest used aircraft starts at ten times that, given that the mechanical tolerances for aerospace are more stringent than for land-based cars, and the demand for small aircraft is substantially less.
Further, private aircraft have not enjoyed most of the safety advances seen in motor vehicles. Life-saving features such as airbags and crumple zones are rare, and only a select few planes feature roll cages and emergency parachutes.[43] As most private planes have forward-mounted engines, the lightweight fuselage (skeletal structure) of the aircraft often lacks the structural integrity to prevent the engine from crushing the occupants upon forward impact. The survivability of a crash, therefore, becomes a dubious prospect in many cases – the safety measure, by and large, is to not crash in the first place.
There are debatable causes to this slower progress of innovation in aviation, but what’s certain is that circumstances are changing. Some of these changes involve the greater inclusion of common-sense safety features into light aircraft. Yet other companies have embraced advances in technology that allow flying craft to be redesigned from the ground-up. Some of these are already seen today in the form of quadcopter drones.
As opposed to helicopters that use two or four blades on a single rotor, quadcopters have four rotors on opposite and balanced points, thus removing the need for a stabilizing tail rotor. This makes quadcopters extremely well balanced, which in turn makes them both more maneuverable and easier to fly than traditional aircraft.
The concept of a quadcopter large enough to carry people (if only for short distances) has already been proven to work with today’s materials. The SureFly personal flying vehicle is one example of quadcopter innovation reaching commercial viability.
The Scorpion-3 hoverbike is another recently developed prototype:
These images below show the Ehang 184 personal quadcopter:
While such quadcopters are possible with today’s technology limitations, their capabilities can be extended via graphene’s capacity for structural strength and energy storage. As discussed throughout this writing, graphene is an ultra-strong, ultra-light, and ultra-conductive material that Scarcity Zero can cost-effectively synthesize to effectively unlimited scales.
With it, not only can we store the requisite energy to power a large quadcopter with minimal added weight, we can also integrate the storage medium into the fuselage while ensuring uniformly high strength. This would make quadcopters light and large enough to transport both people and materials.
After tackling energy storage and weight capacity, the next obstacle to building a large quadcopter is a design that makes the quadcopter cost effective enough to work for transportation – ideally in a way that can both fly and drive.
While we don’t have a human-sized solution yet, smaller-scale models have been recently released that demonstrably prove the concept as workable.
Approximately two feet long and made with the same polycarbonate materials comprising bullet-resistant glass, this vehicle can drive over obstacles at speeds exceeding 20 miles per hour and engage flight rotors at a push of a button.[45]
While there are of course obstacles to scaling a smaller prototype to the size of a vehicle large enough to transport people or cargo, they are absolutely solvable as they center on areas that Scarcity Zero gives us the means to address:
- Ultra-strong, lightweight materials at acceptable cost – made possible through graphene and next-generation synthetics.
- Lightweight energy storage mediums – made possible through a graphene-interwoven polymer/polycarbonate fuselage.
- Manufacturing capability – made possible through next-generation additive / 3D-printing, virtual modeling and precision automation.
The actual flight of any such vehicle would be as simple as driving a car. Large quadcopters of 50 pounds or more are already being flown long distances using two joysticks and a first-person video screen – far less than the array of functions necessary to fly a helicopter or airplane. Any serious production of flying vehicles would of course carry more options, safety features and regulatory controls, but those are not obstructions to their delivery. It’s also true that certain automobile drivers might not demonstrate the requisite proficiency to pilot flying vehicles. Besides the application of automated flight controls, these concerns can be addressed through more lengthy training and more stringent licensing requirements. Fundamentally, these aren’t details of potential capability. They’re details of process, that and only.
It’s difficult to overstate how much this could improve how we live and move. The overland distance between destinations “as the crow flies” is always shorter than the meandering roads we have to take today, and that shorter distance can significantly decrease the response time of emergency crews, help deliver rapid aid to areas without road access, and, in the case of drone-sized quadcopters, even deliver consumer goods on-demand.[46]
We are only one technological step away from having personal flying vehicles that are safe, strong and easily flown. Vehicles that can use autopilot programs that already fly and land commercial aircraft today.[47] Vehicles that, like the autonomous cars before them, can be charged via wireless power over municipally integrated renewables and through designated relays – potentially enabling effectively indefinite flight.[48]
Many of us spent our childhoods dreaming of the day when we would see flying cars. The technical capabilities that can be at our fingertips tomorrow make this dream possible, along with all others that have allowed this writing to portray a vision for a future brighter than the one we face today.
The areas it places focus, like many of the possibilities opened up by Scarcity Zero, are simply the beginning. We can extend these advancements to anything we can imagine, from our day-to-day lives, to cutting-edge aerospace that can revolutionize not just travel within our world, but well and far beyond it.[49]
This is the nature of advanced infrastructure as this writing refers to it, and the realization of the future it brings is now at our command. We now have the tools to make this – all of this – real. To manifest a reality where we can leverage technology to provide the abundance, advancement, achievement and ascension we told ourselves was the path we were destined to walk. We now have the tools to build such as a world as a testament to the choices we made in its furtherance, and the promises we made to those who came before who brought us to this singular threshold, where it could be transcended, at long last, by our own hand.
A future worth having. The start of something new. And the next giant leap.